Biological Rhythms: Sleep and Eating Behavior
Additional Video and Readings
Video – Author/Source: Ted Talks; How does your body know what time it is? – Marco A. Sotomayor
Marco A. Sotomayor details how human bodies naturally tell time. Michel Siffre’s 1962 experiments gave rise to an understanding of the Circadian rhythm, and subsequent research determined the hormone cycles that determine our sleep and wake cycles and how our bodies process food and drugs. The field of science studying how our brains keep track of time is called chronobiology. The superchiasmatic nucleus determines the daily sleep and wake cycles, informed by factors such as light and noise, called ‘Zeitgebers.’
Reading – Spielman et al., 2014 – Hunger and Eating
Reading – Steinclass et al., 2019 – Psychiatry Clin N Am – Cognitive Neuroscience of Eating Disorders
Reading Reflection #3 – Tarullo, Ewell, & Garrison, 2023 – Policy Insights from the Behavioral and Brain Sciences – Sleep, Poverty, and Biological Stress: Mitigating Sleep Health Disparities in Early Childhood
Chapter 12: Sleep and Circadian Rhythm
Sleep is such an important part of our lives that a lack of it strongly correlates with negative outcomes on nearly every measure of health. People who sleep less than approximately 7 hours a night are at a greater risk for heart disease, stroke, asthma, arthritis, depression, and diabetes. Nearly 20% of all car crashes, both fatal and nonfatal, are attributed to drowsy driving. The cancer research branch of the World Health Organization has determined that disruption of regular sleep is “probably carcinogenic to humans,” putting it in the same risk category as the infectious agents malaria and human papillomavirus (HPV), as well as the biochemical weapon mustard gas. Sexual health is affected by sleep deprivation as well, as men with the worst sleeping habits have significantly lower sperm counts, decreased circulating testosterone, and even testicular shrinkage.
Despite all that we know about the benefits of sleep, sleep is often the first time commitment to get cut, often getting squeezed as people stay awake later while waking up sooner. Consider that the CDC estimates that more than a third of American adults fail to get enough sleep each night. Almost 70% of college students fail to get the recommended amount of nightly sleep, and half of all college students report experiencing daytime sleepiness as a result.
The current medical recommendation is 7-9 hours of sleep each night. But why is sleep so important? It is possible to study sleep using a combination of techniques; the output of sleep studies are visualized on a polysomnogram (pahle-SOM-nuh-gram; somn- is the prefix referring to sleep). Several physiological measures are taken in a polysomnogram, including heart rate, blood pressure and oxygenation level, respiratory depth and pattern, muscle activity, eye movement, and one of major interest to neuroscientists, brain wave activity.
While everyone knows what sleep is, it is useful to try to more precisely define sleep as a biological function. Sleep is characterized by the following:
A decrease in physical activity
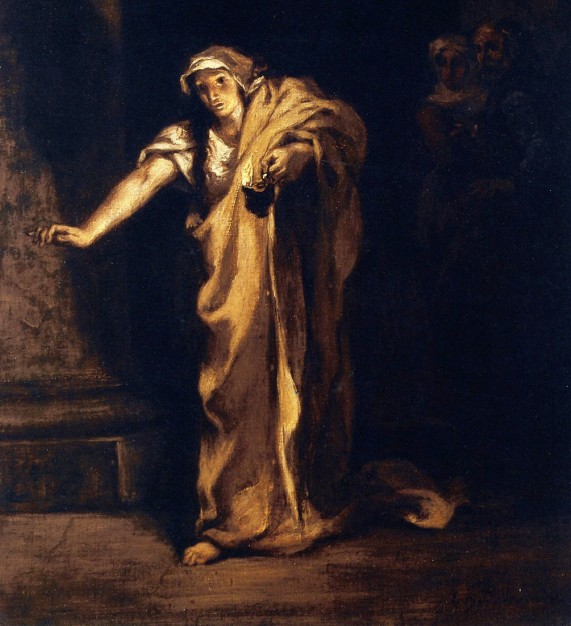
Compared to waking behavior, a person’s physical activity is greatly decreased when they sleep. While asleep, people are relatively inactive, and as a result the body uses about 10% less energy.
This is not to say that people do not completely cease all movement during sleep. It is very common to readjust posture many times in the middle of the night. Some people may grind their teeth together or talk in their sleep, sometimes carrying on full conversations by themselves! About 15% of people have experienced somnambulism, or sleepwalking: full on wake-like behaviors such as navigating down a flight of stairs or preparing a sandwich, performed entirely in the absence of intent of memory recall. Despite these rare occurrences of physical activity, the average movement of the person over a night’s rest is still less than their average activity when awake.
A decoupling from external inputs
When we sleep, our conscious brains are “distanced” from the outside world. Sleep causes a heightened threshold for detection of stimuli, so we do not receive the same magnitude of inputs from our sensory systems as when we’re awake. This is why someone else might have to talk loudly or even shake you physically to wake you up.
Changes in brain wave activity
Sleep was once thought to be a period of time characterized by low brain activity. After all, the person looks like they are not moving. Shouldn’t brain activity be reflective of that decreased state of activity? With the advancement of EEG technology in 1924, and the rise of sleep laboratories in the 1970s, scientists who studied brain activity noticed that, at different times throughout the night, the brain of a sleeping person was very similar in activity to the brain of an awake person!
12.1 Phases of sleep
Each night when we go to sleep, our brains undergo a very stereotyped pattern of activity changes. At times, neurons in the cortex exhibit synchronized patterns of firing. And at other times, cortical activity looks very similar to an awake brain.
We can divide sleep roughly into two different phases depending on one of the first physiological measures that sleep scientists studied: eye movement. A study published in 1953 used a device to detect eye movement while a person was asleep. Interestingly, they noticed that at some points in the night, usually first occurring around three hours after falling asleep, the patient’s eyes would dart rapidly and jerkily back and forth, a pattern of activity that the University of Chicago researchers called rapid eye movement (REM). This first period of REM activity lasted for about 20 minutes, after which the eyes would stop moving again. This activity pattern repeated every hour or two for the rest of the night. They used eye movement to separate sleep into two phases: REM sleep, and nonREM sleep (NREM sleep).
In addition to eye movement, they observed and measured other physiological behaviors. Respiration rate and heart rate both increased during the REM phase of sleep and dipped during NREM sleep. They also (rudely) woke up patients throughout the two phases of sleep, and found that patients were more likely to recall dreams with visual imagery if their REM sleep was interrupted. Those woken during NREM sleep were less likely to recall dreams, hinting that dreaming is more likely to happen during REM sleep.
While eye movement could differentiate between two phases of sleep, another common diagnostic technique, electroencephalography (EEG), could further subdivide NREM sleep. EEG measures electrical activity at the scalp, detecting the firing of large numbers of cortical neurons. Using EEG, scientists discovered three distinct NREM phases based on neuron activity patterns: NREM1, NREM2, and NREM3 sleep. Currently, readings collected via EEG are considered to be the gold standard for measuring the stages of sleep.
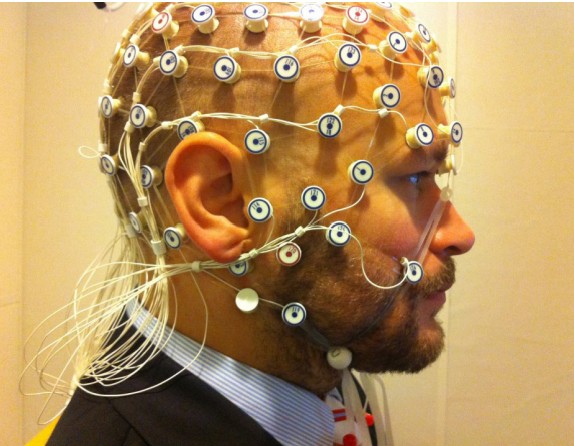
But before we describe EEG traces while asleep, we should describe the EEG of a person who is awake. Usually, the awake EEG is dominated by high-frequency waves, falling in the beta band range of frequencies: between 13 and 30 Hz. The proportion of neurons firing at the beta frequency increases with attention and mental activity: When a person is concentrating on a task, such as reading a textbook, the beta wave frequency dominates.
NREM1 is the earliest stage of sleep. It’s also described as relaxed wakefulness, drowsiness, or light sleep. During NREM1, a person’s muscles are still somewhat active, their eyelids may open and close every so often, and they may still respond to questions. In NREM1 sleep, the beta frequency amplitude decrease as the slower frequency alpha waves (8-13 Hz) increase in amplitude. Late in NREM1, theta waves (4-8 Hz) become more prevalent. Basically, the deeper into NREM1 sleep a person becomes, more waves with lower and lower frequencies start to emerge.
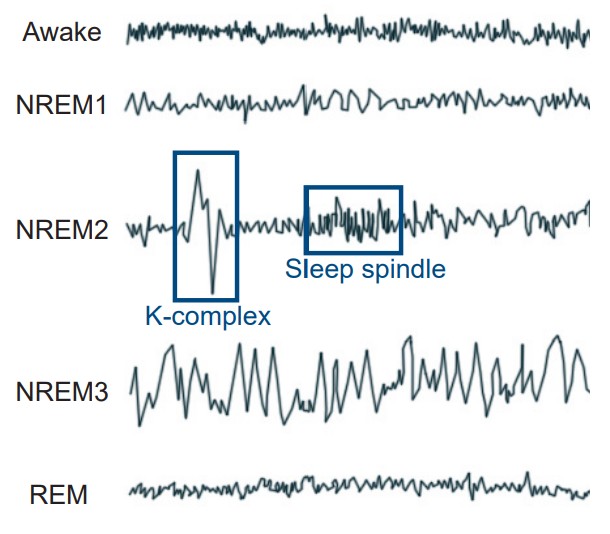
During NREM2 sleep, theta waves predominate. In a healthy adult, about 50% of a night’s sleep is spent in NREM2. NREM2 is characterized by the appearance of two patterns of activity that interrupt theta activity. K-complexes are large amplitude events that are observed about every minute. These are the largest amplitude events in a healthy human EEG. Following a K-complex you may see a sleep spindle, a high-frequency burst of rapid neural activity in the low beta range that lasts for about a second. It is unknown exactly what the function of these sleep spindles are, but some research suggests they may be involved in memory processes or to minimize perception of outside noises, which can help a person stay asleep even in the face of disruptive stimuli.
NREM3 is also called deep sleep. At this phase of the night, a person’s physiological activity drops to its lowest point of the night: heart rate, respiration, blood pressure, and metabolism all reach minimum during NREM3. In this stage of sleep, many of the cortical neurons fire in synchronicity with one another, and the subsequent change in potentials cause large amplitude deflections in the EEG, at the low frequency delta band (1 – 4Hz). Because the delta frequency is much slower than frequencies detected in lighter stages of sleep or wake, NREM 3 is also called slow wave sleep.
The EEG trace of a person in REM sleep is quite the opposite of what is seen in deep sleep. Instead of large amplitude events at a low frequency, the REM brain has a lot of low amplitude events at a high frequency. In fact, the brain in REM sleep has a pattern of activity that is more similar to a person who is awake than asleep! Because of this asynchronous firing activity, REM sleep is sometimes also called paradoxical sleep.
To illustrate the stages of sleep that a person experiences each night, we can use a hypnogram. These charts to plot time on the x-axis, and stage of sleep on the y-axis. Awake is represented at the top, and deep sleep is at the bottom.
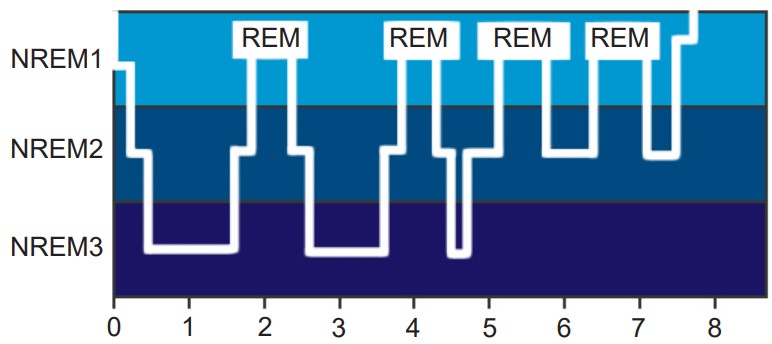
For an average night’s rest, neural activity will fluctuate through the four phases relatively predictably. When a person first falls asleep, they will move from NREM1 down through NREM2 then NREM3, before coming back out of deep sleep progressively back to NREM1. After NREM1, they may enter REM sleep before transitioning back through the stages to NREM3 again. This cycle of activity repeats roughly every one and a half hours.
People spend a larger percentage of each cycle in deep sleep and very little time in REM sleep early in the night. On the other hand, in the last few cycles before waking up from a full night’s rest, people spend a larger percentage of of each cycle in REM sleep, and almost no time in deep sleep.
12.2 Why do we sleep?
All organisms that we know of experience some type of sleep. But we still haven’t figured out exactly why animals sleep. Here, we will discuss three theories that have been proposed to explain sleep. None of these theories alone fully explains the complex phenomenon of sleep, and they are not mutually exclusive. The most likely reason we sleep is probably some combination of the following three theories.
Recuperation Theory
The recuperation theory of sleep is centered around the idea that being awake is stressful and exerts a physically demanding toll on the body. The body therefore needs a period of time when energy usage decreases and the body’s natural repair systems can work without disruption. Sleep is how the body “wipes the slate clean” and resets.
Case study: Peter Tripp
In 1959, New York City radio DJ Peter Tripp ran a publicity stunt to raise money for the charity March of Dimes: he stayed awake for 201 hours. Sitting inside a glass booth in the middle of Times Square, Peter played music and broadcasted his experiences across the airwaves. The first night of sleep deprivation wasn’t awful, but the next seven consecutive days and nights were a real challenge, for both Tripp and the doctors who kept an eye on him.
Just a few days in, Peter began experiencing severe psychological side effects. Tripp developed intense paranoia: He mistook his psychiatrist, who was wearing all black, for being the undertaker here to collect Peter’s body for a funeral. Tripp even began hallucinating, seeing spiders and rodents in his clothes – prompting him to strip naked and run into the street screaming.
Some believe the long-term sleep deprivation severely affected his brain beyond this temporary psychosis. After the experiment, Tripp was involved in a huge commercial bribery scandal, lost his job, and got divorced (Of course, life has many variables, so we’ll never be completely sure of the precise effect long-term sleep deprivation may have had on his relationships and decisions.) Today, the Guinness Book of World Records no longer allows people to compete for the prolonged wakefulness record due to health concerns.
Evidence for the recuperation theory comes from experiments tailored around the idea of looking at what happens when a person doesn’t get sufficient sleep. As anyone who has ever pulled an all-nighter can attest, a single night of sleep deprivation often leads to significant psychological changes, including anxiety, irritability, and mood swings. Staying awake even longer than 24 hours can cause more severe changes in mind state, such as temporary psychosis, hallucinations, or delusions.
The recuperation theory is supported by several pieces of evidence, three of which we will discuss.
1. Enhanced metabolic cleaning during sleep.
When you’re awake, your cells produce several biological waste products during cellular respiration. These chemical byproducts can be potentially toxic to the body when they accumulate. The glymphatic system, a sort of cellular rinse that floods the extracellular space with CSF, clears these cellular waste byproducts when they accumulate in the brain. When we sleep, the extracellular space expands by about 60%, which increases the ability for CSF to penetrate deeper into the brain tissue.
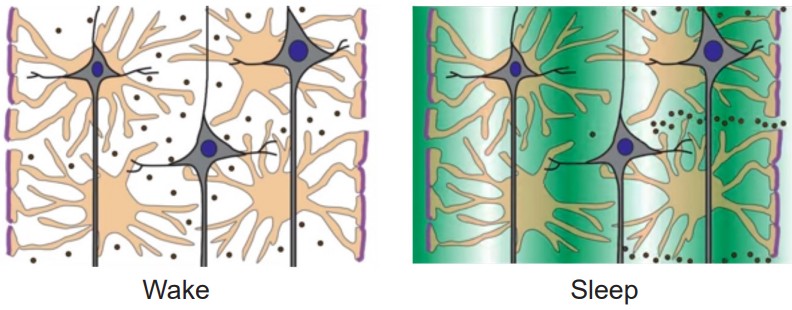
One byproduct of interest is the molecule beta-amyloid, a protein that exists in the healthy brain. But, accumulations of beta-amyloid are found at high levels in the post-mortem analysis of brains from patients with Alzherimer’s disease. During sleep, the glymphatic system’s “rinsing” activity washes away beta-amyloid from the interstitial space and degrades the protein.
2. Immune system function improved with sleep.
Sleeping more enhances your ability to fight pathogens. Sleeping for fewer than 6 hours per night increases the likelihood of catching a variety of transmissible illnesses, such as cold, flu, or gastroenteritis. Sleep increases the effectiveness of vaccines, and each hour of sleep over 6 hours increases said effectiveness by about 50%.
3. Increased production of growth hormone during deep sleep.
Most cells of the body are turned over regularly as cells die and get replaced. One of the signaling molecules that encourages the replacement process is growth hormone (GH), which enhances cellular repair, muscle and bone growth, and protein synthesis. Normally, throughout a 24- hour day, GH is produced and released throughout the body by the hypothalamus. The largest wave of GH production occurs early in sleep, during NREM 3 sleep. During this huge burst of GH release, a person’s circulating plasma GH concentration may be 10 times higher than at baseline.
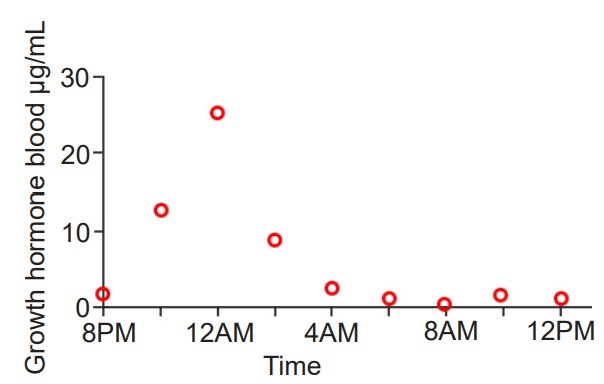
While the support for the recuperation theory is generally true for almost all people, there are about 1% of people who seemingly gain the restorative benefits of sleep, even with fewer than 6 hours of sleep each night. They may wake up at 4:30 in the morning feeling completely refreshed. And yet, despite getting so little sleep, these short sleepers have similar health outcomes with respect to body mass index and psychiatric measures such as depression and overall optimism.
Something about the circadian rhythms of these short sleepers allows them to “maximize” their sleep efficiency. Many have very short sleep latencies, meaning they fall asleep within minutes after lying down – as quickly as someone with narcolepsy. They also spend a larger percentage of their night in deep sleep and REM sleep while minimizing NREM1 and NREM2.
Evolutionary Adaptation Theory
The evolutionary adaptation theory is the idea that animal sleep patterns are different across species for reasons that most efficiently benefit each animal. Over millions of years of evolution, individuals with the most ideal sleep patterns have an advantage, and their sleep habits will be selected for in the following generation.
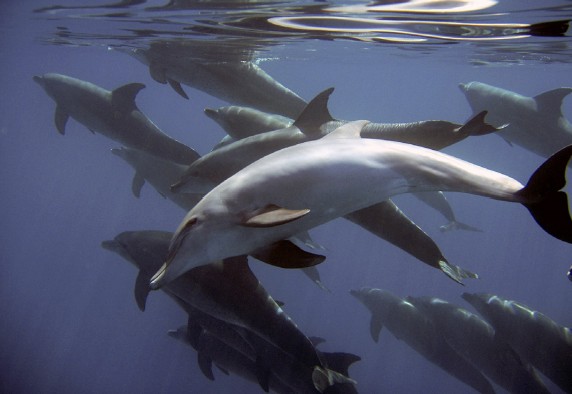
For example, consider humans. As an animal highly dependent on light and the visual system for navigation and accurate performance of tasks, the dark is a very dangerous time to be active. The risks of wandering off a cliff, running head-first into a tree while escaping a predator, or eating a wrong-colored poisonous berry would all be elevated in the dark. We benefit from behaviors that minimize those risks, such as inactivity until the sun rises. During this inactive period, sleeping decreases our metabolism and our body’s need for energy.
Humans are just one animal that has an evolutionarily fine-tuned sleep pattern. If you look across the animal kingdom, you’ll find all varieties of sleep behaviors that are best fitted to the needs of the individual species. In the wild, for example, dolphins are generally prey. They evolved with the ability to put one half of their brain to “sleep” at a time, allowing the “awake” half to keep an eye out for potential predators. Small prey animals, like squirrels, are faced with the threat of being attacked at night. For them, remaining very still, quiet, and hidden improves their survival. Tigers, the top alpha predators in any ecological niche, have almost no predators to hide from, allowing them the luxury of sleeping up to 20 hours a day.
This evolutionary adaptation theory argument has a major weakness, however. In almost all animals, sleep represents a period of time when an organism’s ability to use their sensory organs to detect the hallmark signs of an approaching predator, like the flurry of feathers from a hungry owl or the soft padding of a wolf footsteps, decreases drastically. For an animal that can’t hide very effectively, sleep represents a period of vulnerability, as they would be unable to sense incoming threats.
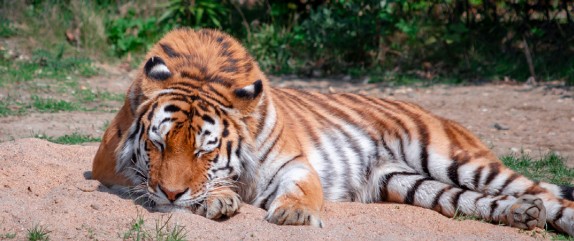
Brain Plasticity Theory
The brain plasticity theory suggests that the brain needs some period of time for critical changes to occur. During sleep, circuits in the brain undergo consolidation processes that are important for memory formation. For example, academic performance and examination grades worsen as a person’s nightly sleep decreases.
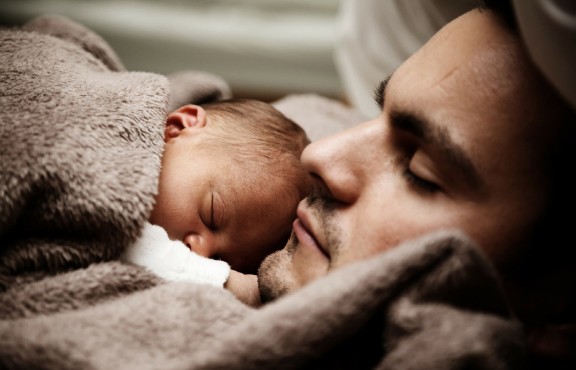
Both the REM and NREM3 phases of sleep are important for different types of memories, and studies suggest that declarative memory, pieces of information about facts, benefits more from slow-wave sleep while procedural memory, the learning of motor skills, is enhanced by REM sleep. Although the exact mechanisms about how sleep improves memory are unknown, we theorize that brain activity during sleep helps move memories held in “temporary” areas into areas of stable, long-term storage.
The evidence in support of this theory starts with looking at the brains of newborns. When you were first born, during those first few weeks of life, you slept close to nearly 70% of the day, almost 17 hours! At this point in your life, your brain starts to experience all sorts of new sensations: your eyes detect visible light for the first time, the skin feels the air blow past it, and the ears sense new frequencies and combinations of sound waves. As a result of these stimuli, scientists hypothesize that your brain undergoes as many learning events as rapidly as possible. This rapid learning helps you remember what you learned each day so you can respond to your environment as you grow up.
12.3 The circadian rhythm
Almost every living organism that we know of on Earth exhibits some sort of cyclic pattern of activity that closely matches the rising and setting of the sun. The discovery of 24 hour patterns of behaviors began in 1729 with the French scientist Jean-Jacque d’Ortous de Mairan, who documented the movement of the Mimosa pudica plant. This unique organism was chosen since the plant exhibits heliotropism, light-seeking movements. In particular, this plant opened up the leaves in the daytime to capture sunlight, then closed at night, minimizing predation. When the plants were put into a dark room with no exposure to sunlight, to his surprise they still opened and closed their leaves in time with the clock. Mairan concluded that the plant did not change its behavior in response to light, but rather in response to some internal 24-hour clock. His work laid the foundations for future chronobiologists, scientists who study day-night dependent periodic phenomena in living beings.
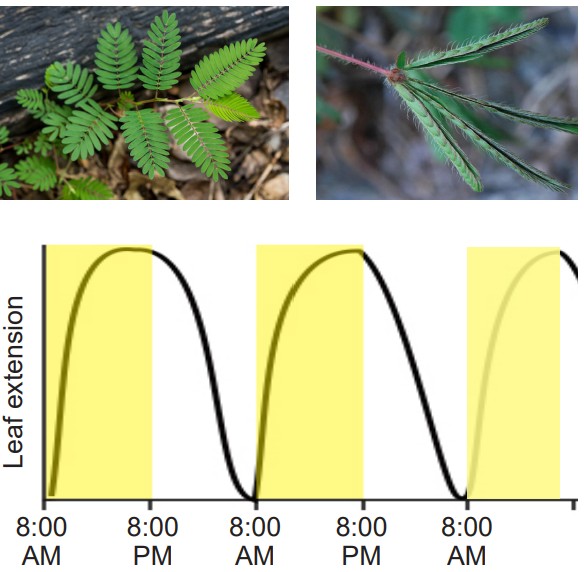
Any behavioral or physiological measure that intrinsically cycles on a 24-hour pattern is said to be a circadian rhythm. The word circadian comes from the Latin words circameaning “around”, and diem meaning “day”. Compare this with an ultradian rhythm, any cycle that is faster than 24 hours, such as the cycling between deep sleep and REM sleep every 90 minutes. Alternatively, compare with infradian rhythms, patterns that are longer than 24 hours, like the 4-week-long human menstrual cycle.
Although we mostly think of the circadian rhythm in the context of sleep and wake, many other physiological measures fluctuate reliably throughout the day. Blood pressure peaks at 11 AM, making the morning the time with the highest risk for cardiac events. Body temperature dips late in the evening, putting the body into a low-energy state that helps promote sleep. Withdrawal reflexes peak around midnight, hunger-driving hormone production rises before lunch and dinner, attention is usually highest in the morning – all manner of behaviors that rise and fall depending on the time of day can be said to be a part of a circadian rhythm.
Bizarrely, even organisms with a life span shorter than a full day still exhibit 24 hour cycling patterns of circadian rhythm-like behaviors. Cyanobacteria, or blue-green algae, are capable of a process called nitrogen fixation, where they convert atmospheric nitrogen into organic compounds like amino acids. These organisms are capable of asexual reproduction every 6 hours or so, but they still have nitrogen fixation patterns that align with daily patterns of light and dark.
Circadian rhythms on a behavioral level
Luckily, a person’s circadian rhythm is not permanent. Anyone who has traveled overseas to a different time zone for more than a few days has experienced that uncomfortable sensation called jet lag, where a person experiences psychological symptoms such as difficulty concentrating and mood swings, and physical symptoms like daytime fatigue, insomnia, and gastrointestinal distress (nausea, constipation, or diarrhea). Jet lag happens when there’s a mismatch between the internal environment and the signals that the brain receives from the outside world. If you flew east from Chicago to Cairo, for example, your circadian clock will be off by 7 hours. When your internal “Chicago” clock is telling you to start getting sleepy around 11 PM, the sun will be rising in Cairo, as the locals are starting to wake up. You may be eating when you’re not hungry or laying down in bed when you’re not sleepy, and this mismatch contributes to jet lag.
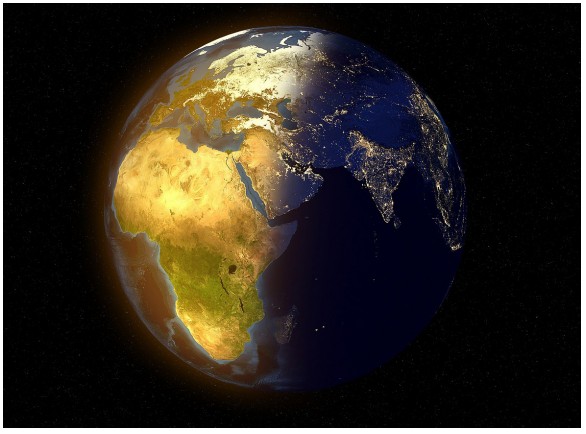
However, with a few days of adjustment, you will be able to overcome jet lag. You will start sleeping as the sun sets, you will get hungry at the same time as the Egyptians, and your physiological measures will start to align with your time zone. This adjustment is only possible because our circadian rhythms are entrainable, meaning they are able to change and fit the surroundings. Our circadian rhythms entrain in response to zeitgebers, the German word for “time givers”: environmental cues, such as increased light exposure when the sun comes up, or social cues, such as increased sensory input from heightened activity of the people around you. A rise in the neurohormone melatonin is an important signal that contributes to helping the brain entrain, which is why taking a melatonin supplement late at night when in a new time zone may help someone get over jet lag more quickly.
If sunlight is a trigger that helps a person entrain their circadian rhythm to new environments, what would happen if a person is completely isolated from sunlight? In other words, what does a free-running circadian rhythm look like? One of the early documented case studies addressing this curiosity was conducted by a French cave explorer named Michel Siffre. In 1972, Siffre (voluntarily) spent six months deep in a Texan cave to evaluate what would happen to a person completely isolated from zeitgebers. At the end of the experiment, he found his circadian cycle was much longer than 24 hours, and very unpredictable – some of his so-called days would consist of being awake for 36 hours and asleep for 12.
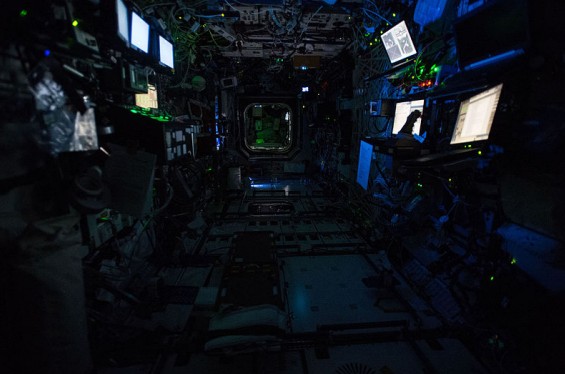
A more rigorous scientific study, conducted in a group of people living in an underground bunker with unchanging lighting conditions for several days estimates the typical free-running circadian cycle to be close to 26 hours. In other words, a person absent from external cues begin to fall asleep and awaken 2 hours later each day.
Free-running circadian disruption is a potential issue for scientists aboard the International Space Station, who experience about 16 sunsets and sunrises per day, since the orbiting space vessel completes one trip around the earth every 90 minutes. In order to minimize the negative effects of jet lag on the researchers aboard, NASA has the inside of the vessel set to an artificial 24-hour cycle. Bright blue LEDs illuminate the cockpit in the “daytime,” while dim, red-shifted wavelengths are used in the evening to induce sleepiness.
Circadian rhythms on a molecular level
The circadian cycle functions on the molecular scale at the level of cellular transcription. In the mid 1980s, the gene period was discovered in the genome of the fruit fly, Drosophila melanogaster. Normal fruit flies are no exception to 24-hour cycles of behavior, as they generally exhibit periods of wakefulness that are paralleled by the rising and setting of the sun. But, if this period gene was mutated, there was an unusual change in the sleeping habits of the flies. Some of them slept on a 29-hour cycle, some had a shorter rhythm at 19 hours, and some had no predictable sleep-wake pattern whatsoever.
Later, it was discovered that another gene was related to the cycle of sleep and wake, called timeless. This gene codes for a protein called TIM, which interacts closely with the protein coded by period, the protein PER. When PER and TIM interact with each other, they form a dimer (a pair of molecules) with the ability to enter into the nucleus, bind to a specific sequence on the genome, and prevent further transcription of both PER and TIM. Therefore, the paired proteins function as a negative feedback regulatory system.
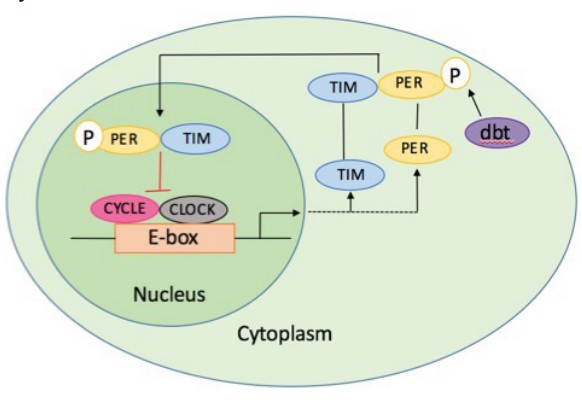
The TIM protein, however, is degraded by light, so during the daytime, the concentration of TIM in the cell is very low. As a result, the PER protein is left by itself, where it can no longer repress transcription. The cells, without the active repression of transcription, then proceed to create more protein. Once night falls, light no longer breaks down TIM, and TIM begins to accumulate again, forming the dimer with PER, which prevents further protein transcription. The cycle repeats itself the next morning.
Since behavior can be driven or modified based on protein levels, the capacity for an organism to change transcriptional activity on a 24-hour cycle suggests that genetic level changes can possibly influence the activity of the whole organism. These gene transcription-level changes were discovered in Drosophila in 1990, and three scientists, Hall, Rosbash, and Young, were recently awarded the 2017 Nobel Prize in Physiology or Medicine.
12.4 Neurochemical signals
Many of the neurotransmitters that the brain uses for signaling are capable of modifying some aspects of sleep. For example:
• Glutamatergic signaling is heightened during the awake state, and many glutamatergic neurons increase their activity during REM sleep.
• Drugs that increase the action of GABA by acting as positive allosteric modulators are used as sedatives and sleep aids.
• Norepinephrine, acting through increasing activity of the sympathetic nervous system, enhances alertness.
Be aware that many neurotransmitters can affect sleep behavior. However, we are only going to describe the function and actions of three sleep-related neurochemicals.
Adenosine
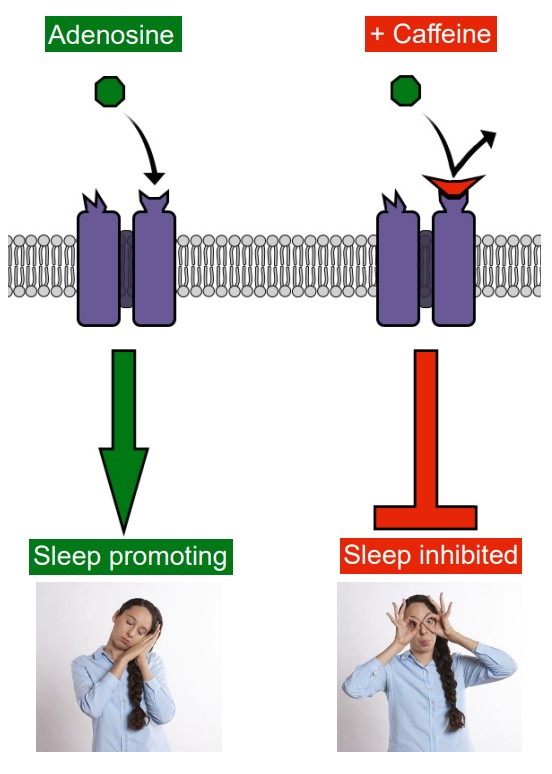
Adenosine is a molecule that has a variety of functions in the body. In addition to being one of the four main building blocks of DNA (the “A” of the A:T G:C base pairing combinations), it acts as a signaling molecule in the body that is involved in inflammation, the immune response, and modulation of heart rate.
It is also used as part of the molecule that stores cellular energy: ATP, or adenosine triphosphate. Each molecule of ATP has phosphate bonds that release tremendous energy when the bonds get hydrolyzed (broken apart). Vesicles, in addition to containing neurotransmitters, have many molecules of ATP. Throughout the day, as the body uses up cellular energy, there is an increase of adenosine as a result. Therefore, as our energy consumption increases, so do adenosine levels, thus signaling to the brain that we are sleepy.
If you are able to chemically block the action of adenosine, you can stave off sleepiness, increasing alertness and ability to focus. Odds are good that you have used a psychostimulant to block adenosine signaling this morning, or are drinking some right now. Caffeine, for example, is an adenosine receptor antagonist, and is the world’s most popular unregulated psychostimulant drug. Other common adenosine receptor antagonists include theobromine and theophylline, both of which can be found in tea and chocolate. They are chemically very similar to caffeine and adenine, part of the chemical structure of adenosine.
Melatonin
Melatonin is an endogenous hormone that helps the brain regulate the sleep-wake cycle. Melatonin is produced by a single gland in the brain called the pineal gland, so named for its pinecone-like shape. Specialized cells in the pineal gland convert the amino acid tryptophan into melatonin, which is then secreted into the bloodstream. Increased melatonin levels helps to signal the body to prepare for sleep.
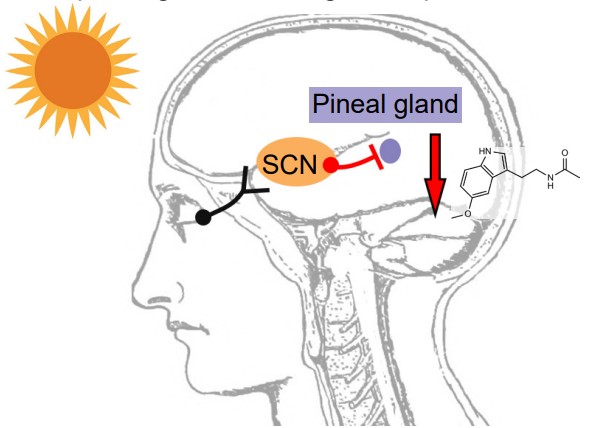
The production of melatonin is heavily dependent on exposure to sunlight. While some of the cells in the retina are responsible for passing specific visual information into the brain, other cells (such as the photosensitive retinal ganglion cells) communicate whether or not it is daytime. These cells send their axonal projections separately from the optic nerve that sends most visual information. Rather, they project through a pathway called the retinohypothalamic tract (RHT), synapsing on a clump of cells in the hypothalamus called the suprachiasmatic nucleus (SCN). In turn, these cells of the SCN send inhibitory projections to the pineal gland. In the day time, the SCN tonically inhibits activity of the pineal gland, resulting in low production of melatonin. But when daylight starts to decrease, the RHT sends a weaker excitatory signal onto the SCN, which allows increased pineal gland activity.
Half-life
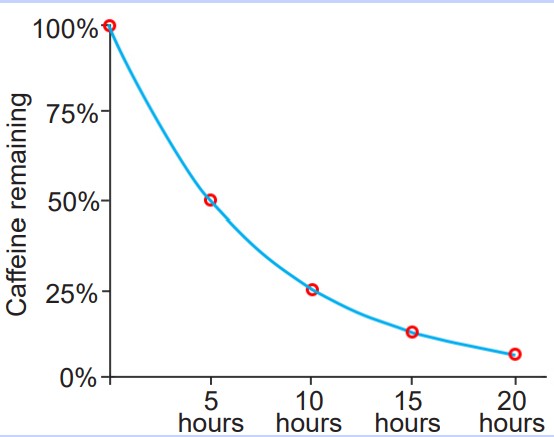
Exogenous substances that enter the body usually get degraded over time through natural enzymatic processes. The half-life, also written as t1/2, is the time that it takes for the concentration of the substance to be degraded to one half of what it originally was.
The half-life of caffeine is about 5 hours. This implies that if you drink a full cup of coffee at 2 PM and plan on going to bed at midnight, the caffeine in your bloodstream is similar as if you just chugged a fourth of a cup of coffee before trying to sleep.
The half-life was originally used to describe the decay of radioactive atoms, but has also been adopted by biologists and pharmacologists. It is safe to say that after 5 half-lives, the substance has effectively been eliminated from a clinically relevant perspective.
Light exposure is the main environmental influence that decreases melatonin levels. But, not all wavelengths of light are equally potent at dampening melatonin production. The shorter wavelengths of light, down in the violet-blue range, are much more efficient at activating the RHT compared to longer, yellow-red wavelengths. Increased RHT activation leads to decreased melatonin production, which can delay the onset of sleep. Fluorescent or LED lighting and digital devices, such as computer screens and cell phone screens, use blue wavelengths of light. Therefore, the best advice to optimize your sleep habits is to eliminate exposure to all digital devices about one hour before your intended bed time.
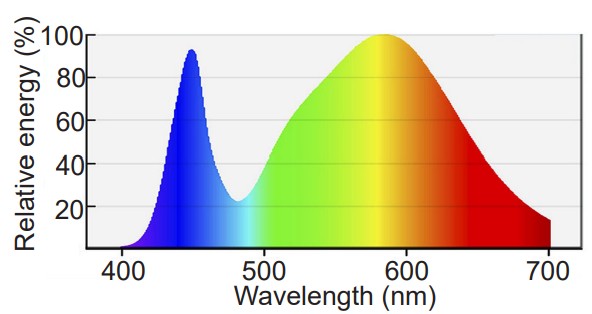
Histamine
Histamine is a small signaling molecule that has a variety of functions. In the body, histamine mediates the sensation of itch, participates in the inflammatory response, and activates the immune system. In the brain, histamine is a neurotransmitter that acts as a prowakefulness signal (the opposite of adenosine and melatonin, which both increase drowsiness.)
Most people understand the role of histamine in the context of allergies. Seasonal allergy sufferers often take a histamine antagonist (antihistamine) to decrease the severity of allergen exposure. Many antihistamines warn against operating heavy machinery while taking these drugs, since drowsiness is one of the major side effects. Newer generations of antihistamines are more effective at minimizing drowsiness, so they often advertise “non-drowsy” on the packaging.
12.5 Brain structures involved in sleep
When scientists use an EEG to measure electrical activity, they look at the activity of neurons in the cortex, the outermost layer of brain cells. However, sleep and wake behaviors are driven by the action of cells that lie buried deep within the phylogenetically older areas of the brain. The signals that originate here communicate broadly throughout the rest of the brain, and these signals are the ones that cause us to sleep or wake.
It is not just a single part of the brain that controls sleep behavior, but most likely a network of communication activity between different areas. Here, we will only address a few of the brain areas that are heavily involved with sleep.
Hypothalamus
Early studies on the role of the hypothalamus in sleep began with Viennese neurologist Constantin von Economo. He described a series of patients with a disease called encephalitis lethargica in 1917. In his observations, patients presented with one of two sets of symptoms. Some had progressive lethargy, starting with drowsiness, moving to extended sleeping periods, worsening to coma. On the complete opposite end of the spectrum, some patients had severe insomnia – a clinically significant difficulty with falling asleep.
When von Economo performed the autopsy on the patients, he found very specific injuries in the hypothalamus that correlated with symptoms. Among those with persistent sleepiness, the posterior hypothalamus was severely damaged. Von Economo concluded therefore that the posterior hypothalamus contained structures that are needed for maintenance of wakefulness in the healthy individual. In the patients with insomnia as their main symptom, their anterior hypothalamus was injured, leading von Economo to conclude that this area was important for promoting sleep.
Clinical connection: Encephalitis lethargica
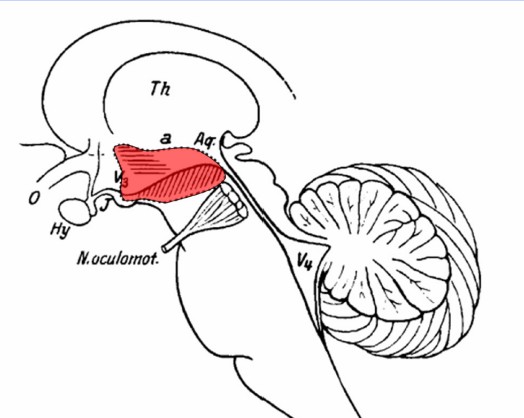
In the midst of World War I, a strange disease of unknown origin called encephalitis lethargica ravaged the globe. A worldwide pandemic, an estimated five million people were affected. About half of the patients died from early stage symptoms, and those who survived often failed to recover fully.
Encephalitis lethargica was also called “sleeping sickness” because of the symptoms that patients experienced: a lack of energy, extreme muscle weakness, and loss of all desires. Neurologist Oliver Sacks described these patients as being “insubstantial as ghosts, and as passive as zombies.”
Today, cases of encephalitis lethargica are extremely rare. The cause of the disease was never figured out, but one theory suggests that malfunctions in the immune system are related to the onset of the disease, since a major outbreak appeared in the wake of the Spanish flu pandemic of 1918.
Von Economo’s findings represented a shift in the way scientists thought of sleep. Most researchers believed that sleep was brought on simply by an overall decrease in brain activity. However, his discovery of hypothalamic localization of a “sleep center” demonstrated that for normal sleep to happen, certain areas in the anterior hypothalamus actually need to increase their activity.
The hypothalamus can be subdivided further into populations of neurons that have previously been addressed in some context. The suprachiasmatic nucleus (SCN), the neurons that receive light information via the retinohypothalamic tract and help regulate melatonin release, is part of the hypothalamus. Elsewhere in the hypothalamus is the tuberomammillary nucleus, the major site of neuronal production of the wakefulness signal histamine. The lateral hypothalamus has neurons that produce the pro-wakefulness signaling molecule orexin (sometimes also called hypocretin), and these neurons are lost in people with severe narcolepsy.
Reticular formation
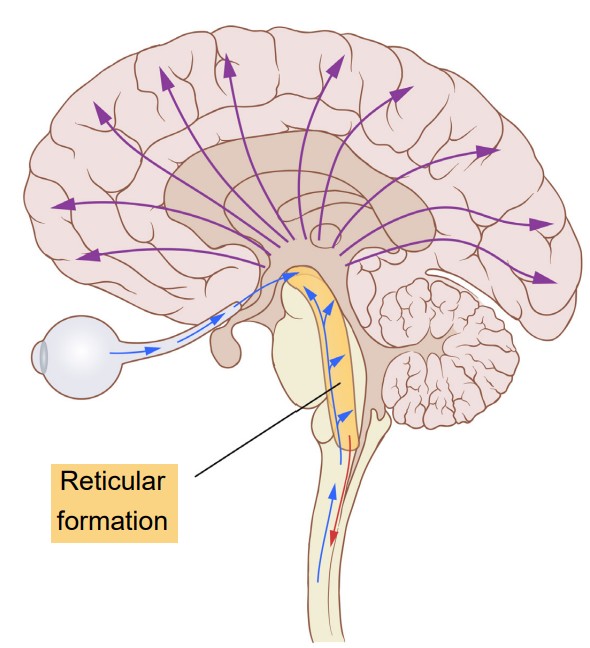
The reticular formation is found in the brainstem. Like a large net of interconnected clumps of neurons, it is difficult to anatomically classify the structures of the reticular formation, since they do not have a clearly defined border or boundary. Activity of these neurons contribute to a variety of behavioral states, such as alertness and consciousness. The reticular formation is vulnerable to ischemia, lesions, or physical trauma. Often, severe injuries may result in a loss of consciousness or coma.
Information flow through the reticular formation passes in the upwards (towards the cortex) and downwards (towards the body) directions. The upward pathway, also called the ascending reticular activating system (ARAS), receives inputs from all the sensory systems before sending wide projections all across the cortex. (For your curiosity, the downward pathway is the reticulospinal tract that is involved with motor control of the skeletal muscles, and is not strongly involved with sleep behaviors.)
12.6 Sleep disorders
Insomnia
Almost everyone has experienced difficulty sleeping at some point in their lives, often as a result of stress or anxiety. For example, it might be difficult to fall asleep the night before a big interview, or you may wake periodically in the hours before an important early morning flight.
There is no strict definition for insomnia. The major clinical symptoms are self-reported measures, such as a dissatisfaction with nightly sleep or a change in daytime behavior, such as sleepiness, difficulty concentrating, or altered mood states. The lack of clear diagnostic criteria makes estimating prevalence difficult, but some guesses put the number of people with insomnia close to one third of the US population.
Common triggers for insomnia include heightened anxiety, stress, or advanced age. It may also be downstream of other diseases, such as Parkinson’s disease, diabetes, depression, or chronic pain conditions. Lifestyle can also be a major risk factor for insomnia, as jet lag and working late-night shifts can disrupt sleep patterns.
We can describe insomnia as acting at two stages. Onset insomnia is defined as a difficulty with initially falling asleep. People with onset insomnia will frequently lie in bed for a long time before finally drifting off. Maintenance insomnia, however, is a difficulty with remaining asleep. People with maintenance insomnia experience many waking events throughout the middle of the night, or they may wake up very early in the morning and be unable to get back to sleep. The two are not exclusive, and people may experience both forms of insomnia in a single night.
The most effective treatments for insomnia begin at the level of behavioral changes. Improving sleep habits, such as minimizing arousal states before bedtime, developing a reliable pattern of sleep-wake timing, eliminating caffeine intake in the afternoon and evening, and increasing daytime physical activity can decrease insomnia. Prescription medications are less preferred for insomnia treatment, since these drugs are more effective at inducing unconsciousness rather than biological sleep. These drugs can also have adverse psychological side effects such as mood swings and depression, and those adverse effects may be more severe than insomnia itself. Prolonged use of prescription sleep medications can lead to a “rebound effect,” causing a person to experience even worse insomnia when they are unable to get sleep drugs. This is called iatrogenic insomnia, and can lead to a cycle of dependence.
Clinical connection: Fatal familial insomnia
While many cases of sleeplessness last a day or two, and some cases are clinically significant and treatable with behavioral changes, a very small fraction of cases of insomnia are incurable and deadly. In people with fatal familial insomnia (FFI), they experience severe insomnia. Some patients stay awake for up to six months at a time. As a result of either the disease or the sleep deprivation, they experience altered mood states, hallucinations, dementia, and eventually death, usually within two years after a diagnosis is made.
The cause of FFI is unknown. There is a strong genetic component associated with it, as it appears frequently within certain family trees. But, there have also been a few cases of sporadic FFI, people with no apparent family members with the disease. One observation in common among people with FFI is significant damage to the thalamus, as a result of misshapen proteins called prions, a similar disease-causing agent that is responsible for mad cow disease.
Sleep apnea
Sleep apnea is characterized by nightly sleep that is frequently interrupted by the inability to breathe (a- meaning none, and pneu referring to wind, air, or breath, as in pneumonia or pneumatic pump). In turn, this lowers blood oxygen levels, causing the brain to wake the person in panic. Each wake event may only last a few seconds, but these disruptions are significant enough to prevent a person from getting the appropriate amount of restorative deep sleep. A person with sleep apnea may wake 30 times an hour, despite having no recollection of waking. The immediate result of sleep apnea is excessive daytime sleepiness, but over long periods of time, sleep apnea contributes to the development of heart disease and an increased risk for stroke.
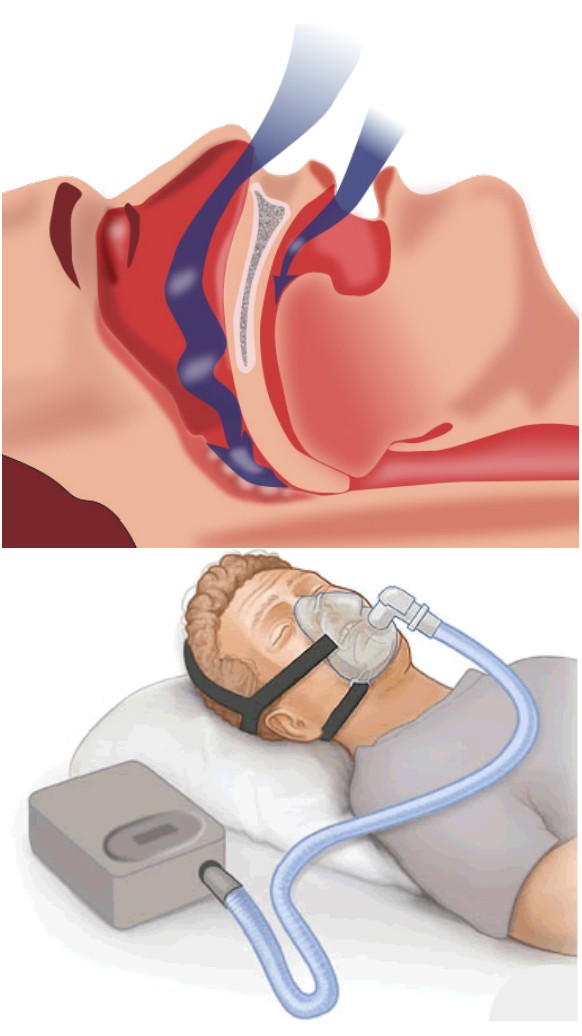
There are two forms of sleep apnea, both of which may be seen in a person with this sleep disorder. Obstructive sleep apnea is the more common of the two. This happens when soft tissue in the back of the throat temporarily collapses, which can decrease or completely block airflow into the lungs. On the other hand, central sleep apnea is mediated by some biological change in the brain that results in a decrease in involuntary breathing patterns at night, possibly due to some damage in the respiratory centers of the brain.
There are several risk factors that contribute to sleep apnea, which are often a combination of genetic and environmental influences. Obesity is a major risk factor, resulting in increased soft tissue mass around the neck and torso, which can increase the likelihood of airway blockage. Advanced age contributes to sleep apnea, as the muscles that keep the airway open weaken and lose tone over time. Exposure to chemical irritants, such as cigarette smoke, contribute to inflammation and increased water retention in the soft tissue, both of which can decrease the size of the airway.
Sleep apnea is most often treated with a portable machine called a continuous positive airway pressure device, or a CPAP device. These machines are basically air pumps that connect to a mask that is worn over the nose and mouth. When used correctly, the CPAP forcibly pushes air into the person’s respiratory system, acting as an external lung. However, the CPAP can be loud and bulky, and the mask must be airtight for the treatment to be effective, making the treatment very uncomfortable. Because of this, CPAPs can cause more difficulty with sleep compared to sleep apnea itself, so they often have a low compliance rate.
Narcolepsy
Unlike the previous two sleep disorders, which result in a deficit of sleep, narcolepsy can be thought of as an “excess” of sleep. More accurately, narcolepsy is inappropriate sleep, and it manifests as frequent sleep attacks throughout the day, each event lasting for seconds or minutes at a time. An estimated 1 in 2000 people experience narcolepsy.
One of the life-threatening symptoms that appears in narcolepsy is cataplexy, which is the sudden weakening of muscle tone that accompanies a sleep attack. A cataplectic attack may cause someone to physically fall over during a narcoleptic incident. Cataplexy often happens during high emotional states, such as excitement.
As with other sleep disorders, changes in lifestyle can improve the course of narcolepsy. Introducing short daily naps can be helpful, as can general good sleep habits (minimal digital device usage before sleep, regular sleep-wake timing, and physical activity). Drugs such as amphetamines (Modafinil) can be used in the daytime to stimulate activity in the CNS, and can be prescribed to treat severe cases of narcolepsy. Some antidepressant drugs can be used to treat cataplexy.
The exact cause of narcolepsy has not yet been identified. However, there are many clues that point to a dysregulation of the signaling molecule orexin produced by cells in the lateral hypothalamus. These neurons die off in people with narcolepsy, but the cause of why the neurons die is unknown. Also, having a genetic predisposition to narcolepsy does not guarantee that a person will experience the symptoms, indicating that there is some combination of genetic and environmental factors that lead to narcolepsy.
Clinical connection: REM sleep behavior disorder
One very rare parasomnia (sleep disorder) called REM sleep behavior disorder (RBD) can cause people to carry out complex, highly coordinated motor actions while they are sleeping, sometimes acting out their dreams as if they were reality. People with RBD are at risk of injuring themselves or others. Their sleep actions may be in response to a violent nightmare, causing them to jump out of bed, kick or punch the air, run through the house, or throw things.
One of the most shocking instances of parasomnia-induced sleepwalking was the 1987 case of Kenneth James Parks, a Canadian man who, in his sleep, drove 23 km to the house of his in-laws and stabbed both of them with a kitchen knife. After his arrest, scientists discovered that his brain activity was highly abnormal during sleep. As a result of the medical examination, the Supreme Court of Canada acquitted him of murder in 1992.
Restless legs syndrome
A person with restless legs syndrome (RLS) experiences frequent unusual sensations in their limbs, such as a tingling or buzzing. Because these uncomfortable sensations disappear with movement, people with RLS often want to move their legs around. Technically, RLS is not exclusively a sleep disorder, since patients experience similar symptoms when they are simply at rest, while sitting and watching TV or studying. But, the sensation happens frequently as a person is lying down in bed, thus delaying the onset of sleep.
RLS is likely underdiagnosed, since it is a disease that exists on a spectrum. Those who are minimally affected probably do not experience significant changes in sleep. Estimates of prevalence of RLS range from 2.5% to 15%. Major risk factors for RLS are iron deficiency and dopamine dysregulation. The exact pathogenesis is unknown, but it does have some genetic component.
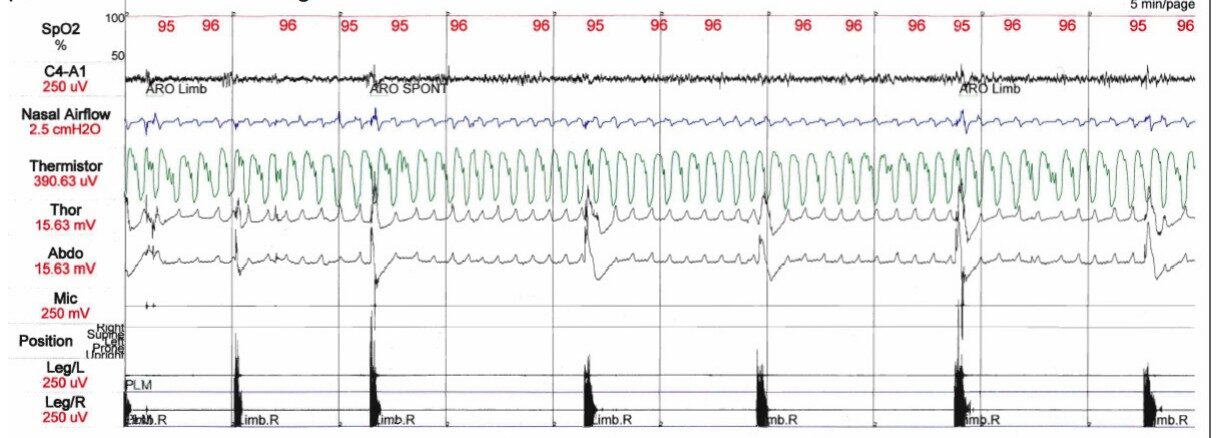
Periodic limb movement disorder
Periodic limb movement disorder (PLMD) is a motor disorder that causes abrupt limb movement such as kicking, flexing, or jerking. Usually, the limb movement is in the legs, but arm movement can also be seen. Since motor activity is actively suppressed during REM sleep, limb movement is most often seen in the first half of sleep when NREM sleep dominates the sleep cycle. Each series of limb activity repeats at about a 30 second interval.
PLMD is different from RLS, since PLMD results in involuntary movements that a person may not be aware of, while RLS related-movement is voluntary. As a movement disorder, the course of PLMD can be modified by dopaminergic drugs. Alternatively, sedatives can help minimize nighttime movement.
Image Credits
12.1 Eugene Delacroix, lady Macbeth sleepwalking
12.2 https://commons.wikimedia.org/wiki/File:EEG_recording.jpg
12.5 modified from https://commons.wikimedia.org/wiki/File:Video_schematic_of_glymphatic_flow.ogv
12.7 https://pixabay.com/photos/dolphins-fish-mammals-delphinidae-380034/
12.8 https://pixabay.com/photos/tiger-big-cat-majestic-noble-zoo-3383616/
12.9 https://pixabay.com/photos/father-baby-portrait-infant-22194/
12.10 Brucewinter [CC BY-SA 4.0 (https://creativecommons.org/licenses/by-sa/4.0)]
Pancrat [CC BY-SA 3.0 (https://creativecommons.org/licenses/by-sa/3.0)]
12.11 Image by Arek Socha from Pixabay
12.12 https://commons.wikimedia.org/wiki/File:ISS-47_The_International_Space_Station%27s_Destiny_Laboratory_
at_%27night%27.jpg
12.13 PranjalAgr [CC BY-SA 4.0 (https://creativecommons.org/licenses/by-sa/4.0)]
12.14 https://pixabay.com/photos/asleep-sleep-bored-tired-nap-3126444/ https://pixabay.com/photos/crazy-fun-personhappy-girl-funny-2840240/
12.15 Davide Gnocchi, Matteo Pedrelli, Eva Hurt-Camejo, and Paolo Parini [CC BY 4.0 (https://creativecommons.org/
licenses/by/4.0)]
12.17 By Lamiot – Own work, CC BY-SA 4.0, https://commons.wikimedia.org/w/index.php?curid=36046024
Kipala [CC BY-SA 4.0 (https://creativecommons.org/licenses/by-sa/4.0)]
12.18 Images are generated by Life Science Databases(LSDB). [CC BY-SA 2.1 jp (https://creativecommons.org/licenses/
by-sa/2.1/jp/deed.en)]
12.19 https://commons.wikimedia.org/wiki/File:Reticular_formation.svg modified by Austin Lim
12.20 https://commons.wikimedia.org/wiki/File:Obstruction_ventilation_apn%C3%A9e_sommeil.svg https://commons.
wikimedia.org/wiki/File:CPAP.png?wprov=srpw1_26
12.21 https://commons.wikimedia.org/wiki/File:PLMD_Polysomnogram.png
measures heart rate, blood pressure and oxygenation level, respiratory depth and pattern, muscle activity, eye movement, and, brain wave activity.
sleepwalking
usually first occurring around three hours after falling asleep, a person's eyes would dart rapidly and jerkily back and forth
a phase in sleep where there is rapid eye movement
a phase in sleep where there is no rapid eye movement
a common diagnostic technique that separates NREM sleep into three phases: NREM1, NREM2, and NREM3
Earliest stage of of sleep; the deeper into NREM1 sleep a person becomes, more waves with lower and lower frequencies start to emerge
NREM2 accounts for 50% of the night's sleep and is characterized by the appearance of K-complexes and sleep spindles
phase of sleep where physiological activity drops to its lowest point of the night: heart rate, respiration, blood pressure, and metabolism all reach minimum during NREM3, also called deep sleep
between 13 and 30 Hz
large amplitude events that are observed about every minute
a high-frequency burst of rapid neural activity in the low beta range that lasts for about a second
a person’s physiological activity drops to its lowest point of the night: heart rate, respiration, blood pressure, and metabolism all reach minimum
REM sleep is sometimes called paradoxical sleep
plots time on the x-axis, and stage of sleep on the y-axis
a cellular rinse that floods the extracellular space with CSF
a protein that exists in the healthy brain
enhances cellular repair, muscle and bone growth, and protein synthesis
pieces of information about facts
the learning of motor skills
light-seeking movements from plants
scientists who study day-night dependent periodic phenomena in living beings
Any behavioral or physiological measure that intrinsically cycles on a 24-hour pattern
psychological symptoms such as difficulty concentrating and mood swings, and physical symptoms like daytime fatigue, insomnia, and gastrointestinal distress; occurs when there is a mismatch between the internal environment and signals from the outside world
ability to change and fit the surroundings
environmental cues, such as increased light exposure when the sun comes up, or social cues, such as increased sensory input from heightened activity of the people around you
a pair of molecules
acts as a signaling molecule in the body that is involved in inflammation, the immune response, and modulation of heart rate; one of four main building block of DNA
adenosine receptor antagonist
an endogenous hormone that helps the brain regulate the sleep-wake cycle
pathway in which retinal cells project
clump of cells in the hypothalamus
the time that it takes for the concentration of the substance to be degraded to one half of what it originally was
in the body, mediates the sensation of itch, participates in the inflammatory response, and activates the immune system; in the brain, a neurotransmitter that acts as a prowakefulness signal
histamine antagonist to decrease the severity of allergen exposure
"sleeping sickness," with symptoms like a lack of energy, extreme muscle weakness, and loss of all desires
the major site of neuronal production of the wakefulness signal histamine
receives inputs from all the sensory systems before sending wide projections all across the cortex
difficulty with initially falling asleep
difficulty with remaining asleep
prolonged use of prescription sleep medications causing a person to experience even worse insomnia than before
severe insomnia eventually resulting in death, either from the disease or sleep deprivation
disease-causing agent that is responsible for mad cow disease
soft tissue in the back of the throat temporarily collapses, which can decrease or completely block airflow into the lungs
mediated by some biological change in the brain that results in a decrease in involuntary breathing patterns at night, possibly due to some damage in the respiratory centers of the brain
air pumps that connect to a mask that is worn over the nose and mouth used to treat sleep apnea
sudden weakening of muscle tone that accompanies a sleep attack
frequent unusual sensations in their limbs, such as a tingling or buzzing